Chemists and engineers in the history and development of plastics
In the 1967 film “The Graduate”, at the party thrown by the Braddocks family for their newly graduated son Ben, a family friend Mr McGuire is anxious to give a word of career advice to him. Those of you familiar with the film will remember that it is indeed only a single word and that word is “PLASTIC”. Would such advice be given today, or perhaps that single word might now be “RECYCLING”?
Plastics have not had a good press of late, because of humanities cavalier attitude to their after use disposal resulting in degradation of the environment by ‘leakage’ into it, particularly the oceans. This currently inadequately managed facet of their use masks in the general view of the public the many benefits that plastics have brought and continue to bring to society. My own career as a chemical engineer has been intimately connected with the petrochemicals industry of which the manufacture of plastics is a major component.
It is ironic perhaps that the first synthetic plastic resulted in a significant environmental benefit. A competition in New York in 1868 to find a manufacturing technique for billiard balls without needing to kill elephants to obtain tusks for the ivory traditionally used resulted in a material called “Celluloid”. Let’s first attempt to define what are plastics. As will be seen later in this text there are so many types, but one source aptly puts it:
“Plastics are man-made materials that can be shaped in almost any form. They may be any colour of the rainbow, or as clear and colourless as crystal. Plastics may have the hardness of steel or the softness of silk. Manufacturers can shape them into long-wearing machine parts or into women’s stockings. The word ‘plastics’ comes from the Greek word ‘plastikos,’ which means ‘able to be moulded’ ”.
Plastics are polymers (long-chain molecules) or combination of such molecules with other substances to give the desired properties. The twentieth century has been the time when modern technological society came about and plastics have been at the heart of that development. The year 1967 when ‘The Graduate’ was made was in an era of rapid expansion of plastics use. Few modern necessities would be possible now without them. They have displaced traditional materials like metal, wood, glass and brick in many applications.
Early plastic materials like celluloid were based on chemical treatment of natural polymeric substances such as rubber and wool or cotton based cellulose. A major breakthrough came with the development of a totally synthetic plastic material by Dr Leo Baekeland in New York in 1905, formed by the reaction of phenol with formaldehyde to produce phenol formaldehyde resin. This very hard material faithfully reproduced the contour of a mould, did not absorb water, did not melt and was a poor conductor of electricity. Commercial production of “Bakelite” started in 1910, with most products going into electrical applications, particularly early black telephones in the USA. Dentures and an ‘improved’ billiard ball were amongst other products made.
The major plastics in use today had their origins in laboratory developments before WWII.
Polyvinyl Chloride (PVC)
Although it was known in the 19th century that vinyl chloride could be transformed into a solid (polymer) it was not until the late 1920’s that several companies in the USA were again working on developing polymers from vinyl compounds.
A major problem that had to be overcome was the rigidity of this polymer, making it difficult to convert into useful products. It is ironic however that this rigidity led later to its major use in the construction of rain and waste water piping. PVC became the workhorse plastic for many military uses so much so that PVC production in the USA rose very rapidly in the early 1940’s. ICI, that renowned major UK chemical producer through all of the 20th century started production of PVC compounds just before WWII using imported polymer from the USA. The company then built a vinyl monomer plant at Runcorn. Notable is its first use in long playing records in 1948.
Polystyrene (PS)
The first recognition of the commercial potential of this material came with a UK patent in 1911. A major problem was the reactivity of the styrene monomer which was difficult to purify and store as it had a tendency to prematurely polymerise during distillation and storage. French researchers provided an answer to this but it was again an US company who were the first to build a polystyrene plant. They were helped by an eminent Russian scientist who emigrated to the USA and filed a key patent in 1927.
Early German uses for polystyrene were in military applications, and the manufacture of articles such as combs, egg cups and drinking glasses. Post WWII it became an important new plastic for injection moulding applications and a component of adhesives, emulsions, and foams. A foamed version was patented in 1944 to give a cheap lightweight material for packing (particularly fragile items) or insulation. In 1960 the first ‘disposable’ foam polystyrene cups were produced. From the late 1950’s the solid clear version began to be used for disposable hypodermic syringes, which have been a major contribution to world health.
Polyethylene (PE)
The discovery of polyethylene (often shortened to polythene) is a most remarkable story and turned out to be one of the most important inventions of the 20th century. Although some work had been carried out by a German chemist just before the turn of the 20th century it was abandoned because of the expense of the process route chosen. The topic was picked up again later in the UK where both the discovery and primary development took place without other international involvement. ICI at Winnington in Cheshire were carrying out basic research into reactions at very high pressure (up to 20,000 atmospheres), when in March 1933 a test autoclave containing ethylene and benzaldehyde at 2000 atmospheres and 170°C was left overnight and on opening found to contain a white material that was later determined to be polyethylene. The experiment could not be replicated and further work discontinued until 1935. It was realised that benzaldehyde did not take part in the reaction and that lower quality ethylene containing trace oxygen catalysed the polymerisation. It was said that it was fortunate that with a little less oxygen nothing would have happened and with a little more, there would have been an explosion.
ICI constructed the first commercial plant, coming on stream in 1939. Virtually all of wartime production was used in electrical insulation applications, primarily for airborne radar installations. The result was that during the early part of the war aircraft operated by Axis powers had much bulkier radar sets than Allied planes. The process was licensed to other companies around the world by ICI and only a few years later it had become the fastest growing synthetic material in the world. The use of polyethylene was soon developed further for the manufacture of flexible piping, wrapping (‘clingfilm’) and domestic articles including washing-up bowls in a variety of colours. The latter was claimed to raise the profile and popularity of plastics in general.
In the early 1950s academic work in Germany resulted in the discovery of a low pressure ethylene polymerisation process using a catalyst, with differing product properties to the ICI process, including a higher density. Thus this product is known as high density polyethylene (HDPE) and that from the ICI/high pressure process known as low density polyethylene (LDPE).
Polypropylene (PP)
The polymerisation of propylene was known from the early 1930’s but it was not until 1954 that the work of Italian chemists led to the ability to manipulate the structure of the polymer molecule. This would lead to another commercially important polymer with a high melting point and good mechanical properties. Its production process is similar to that for HDPE above with similar catalysts. The first commercial plants were built in the late 1950’s and expansion in its use occurred later than with polyethylene, especially post 1970.
Nylon
The practical difficulties of going from initial lab discovery to a practical product on an industrial scale may be illustrated by the 12 years and US$27 million it took for Du Pont to introduce a commercial form of nylon, a strong synthetic fibre, notable as a replacement for silk in stockings, in 1939. In 1941 it contributed greatly to the US effort in the Second World War, replacing silk in parachute fabric. Nylon was also invaluable as strong and rotproof rope and cord. German troops had to wear jackboots because of the lack of laces, as cotton was limited in availability and tended to break. Meanwhile US troops had strong long-lasting laces in their more comfortable and adjustable boots. The name Nylon was thought to be an amalgamation of the words New York and London, recognising the relationship at that time between ICI in the UK and DuPont in the US. Apparently, however there is no truth to this! Although mainly used in synthetic fibres, in solid form it has good strength and self-lubricating properties in mechanical systems such as gears.
Whilst the names of chemists who discovered these polymers are prominent in their history (eg. Karl Ziegler of the Max Planck Institute in Germany, Professor Guilio Natta in Milan, Wallace Carothers of DuPont), nothing significant is written about the engineers who were responsible for designing and building production plants based on the laboratory work. This may have required the building of pilot plants at an intermediate size between laboratory and full production plant. Chemical engineering was not recognised as a separate study subject in many countries, particularly on the continent and the lack of specialists in this discipline was identified in the 1950’s. However, one can surmise that chemists who also became engineers together with those of other disciplines, particularly mechanical engineering, were successful as part of teams to develop these production facilities. This was not achieved without many difficulties in areas such as the safe storage and use of catalysts (many improvements have been made here), safety in overall plant design and polymerisation reaction control. A couple of instances from my own memory illustrate problems here in the 1960’s:
- The LDPE polymerisation reaction taking place in very high pressure (1,000atm plus) piping safely enclosed within a blast wall would sometimes decompose with release of unreacted and reacted ethylene to atmosphere. These ‘decomps’ would be accompanied by black or white smoke but unlike the election of a new pope, there would also be a loud muffled roar as the pressure relief equipment did its job. By the end of the 60’s these events became rare.
- There would occasionally be a runaway polymerisation reaction in the polystyrene plant and the whole reactor contents would solidify. These were referred to as ‘lollipops’ as the cylindrical reactor with hemispherical bottom and stirrer axle once removed resembled the iced product of that name. Removal of the polymer was extremely difficult and defied solvents, pneumatic drills and the advice of ‘Blaster Bates’.
Many other newer polymers have been developed since the war. To illustrate this, a Peugeot 3008 front mask at the Plastic Omnium stand of 2018 Paris Motor Show
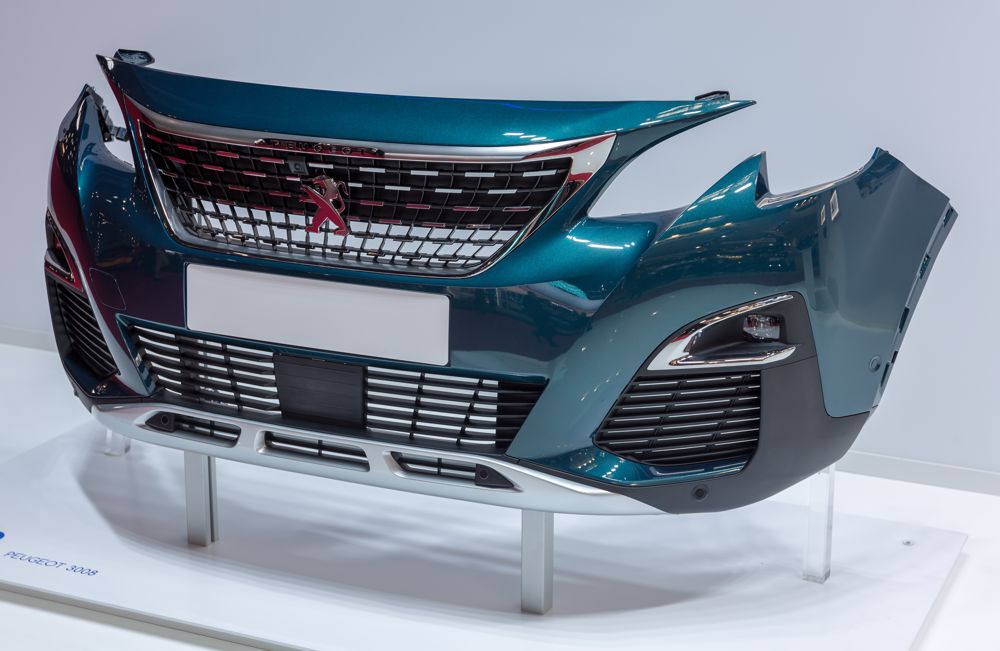
Typically, within a modern car, some 15 different polymers/plastics all with distinct applications are represented.
These plastics contribute more energy efficiency, mainly due to weight reduction, along with corrosion resistance, design flexibility, durability, and higher performance at lower costs. Plastics are lighter and more resilient than metals. As well as those illustrated there are many small plastic items such as: wire insulation, seals, gaskets, seat belt straps, air bags, and light fixtures. Plastics are not there just to keep costs down, they do other things no metal can do. They insulate, absorb vibration, make the car absorb energy from a crash without it doing as much damage to your body. Plastics make the car lighter and that saves fuel.
The drive to also reduce weight in airplanes is aggressive and continuous. Composites play a major part in modern airplanes and the Boeing Dreamliner is no exception in maintaining the decreasing weight trend. Many improvements have contributed to an 11 fold increase in operating efficiency compared to the Douglas DC3 Dakota (dating back to 1936) but composites have had a huge part to play. They are used in the Dreamliner airframe, the engines, and many other components. The use of carbon fibre reinforced plastic and other composites offers weight savings on average of 20 per cent compared to more conventional (and outdated) aluminium designs.
So what are plastics? They are a wide range of ubiquitous materials in our lives today that range from small precision cut contact lenses, transparent tubing and containers attached to hospital patients on life support (much represented on TV during the COVID crisis), materials that save fuel consumed in travel, the computer keyboard I am using to write this text, to the despised single use plastic bag and wrappings. In an article referenced below plastic is quoted as the:
“key enabler for sectors as diverse as packaging, construction, transportation, healthcare and electronics”
It has also been said that:
“Plastic should be a high value material... [It] should be in products that last a long time, and at the end of the life, you recycle it. To take oil or natural gas that took millions of years to produce and then to make a disposable product that last minutes or seconds, and then to just discard it--I think that's not a good way of using this resource. (Robert Haley)”
Nearly all plastics can be recycled and many are, but clearly there is some way to go. Recycling of plastics is a complex subject and deserves a separate article. Even fierce critics do not want a world without plastics, but in the future the current range may be augmented by new polymers and means of production, probably based more on natural biomass feedstocks. This should be accompanied by far more effective recycling and recovery. In any event I forecast that chemical engineers will be at the forefront of the required innovations.
Useful references:
1. ‘The New Plastics Economy’ – report sponsored by amongst others the Ellen MacArthur Foundation
2. British Plastics Federation website: www.bpf.co.uk
3. Plastic Resin Identification Codes (available on www and useful for recycling)