Screaming at dirty water to make it clean
Have you heard of PFAS - per- and polyfluoroalkyl substances? They’re a class of several thousand highly fluorinated organic compounds[2–4] found in water bodies, usually at trace levels[1]. First developed as waterproof coatings for tanks in the 1940s, PFAS have a plethora of uses, spanning from non-stick pans to greaseproof packaging[7], fighting foams, textile coatings and metal plating aids[8]. The high strength and chemically repellent nature of carbon-fluorine bonds[5,6] impart PFAS with resistance to oils, water, chemicals and heat which is far superior other surfactants. The problem is that pollution from PFAS containing products is linked to the development of several cancers, hormonal imbalances, and other serious diseases[9–11]. They do not biodegrade in the environment[12,13] and are poorly removed in conventional waste water treatments[14,15]. In fact, PFAS are so persistent and mobile in the environment that they have impacted drinking water supplies globally and been the subject of several class action lawsuits, such as that described in the true-to-life movie “Dark Waters”.
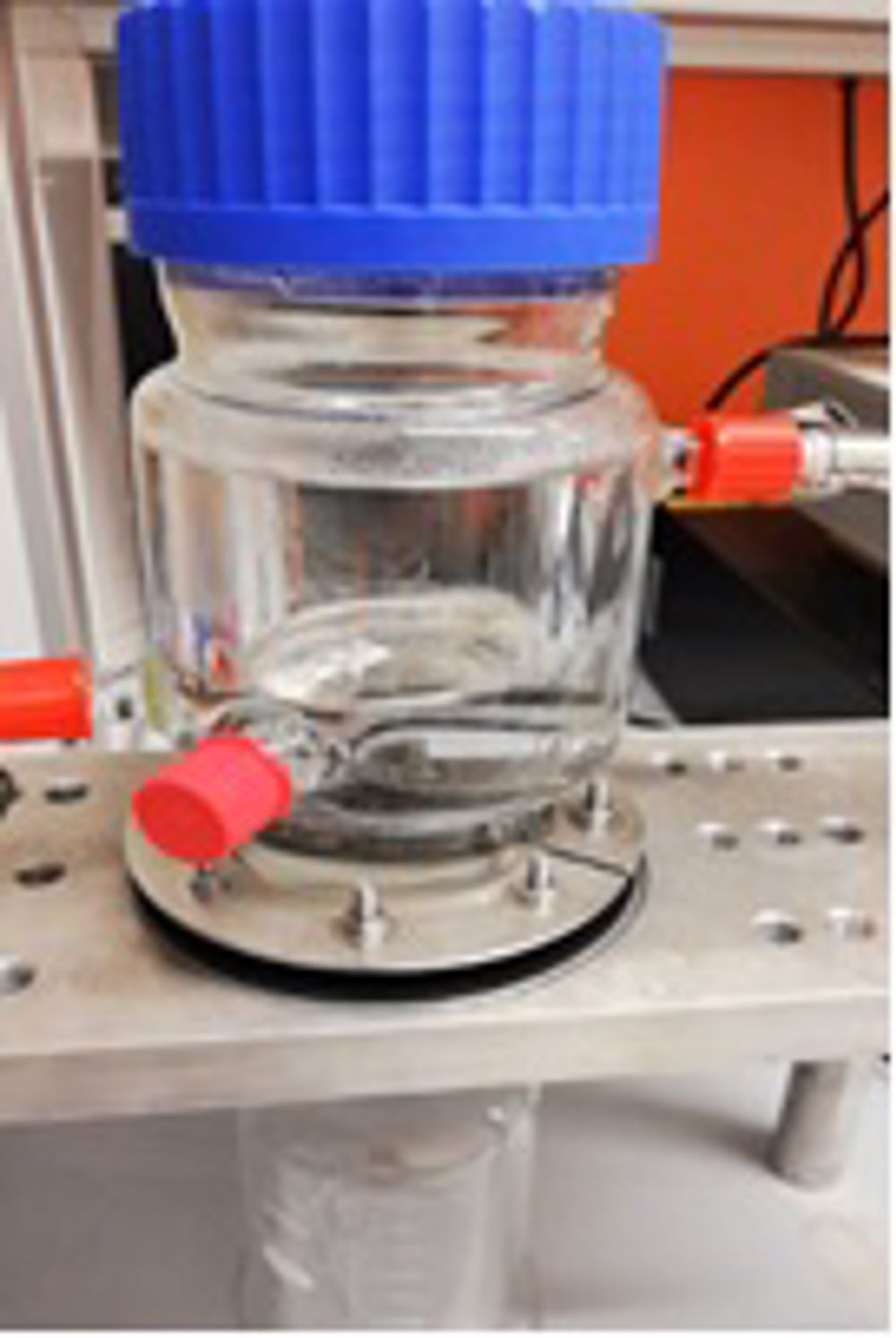
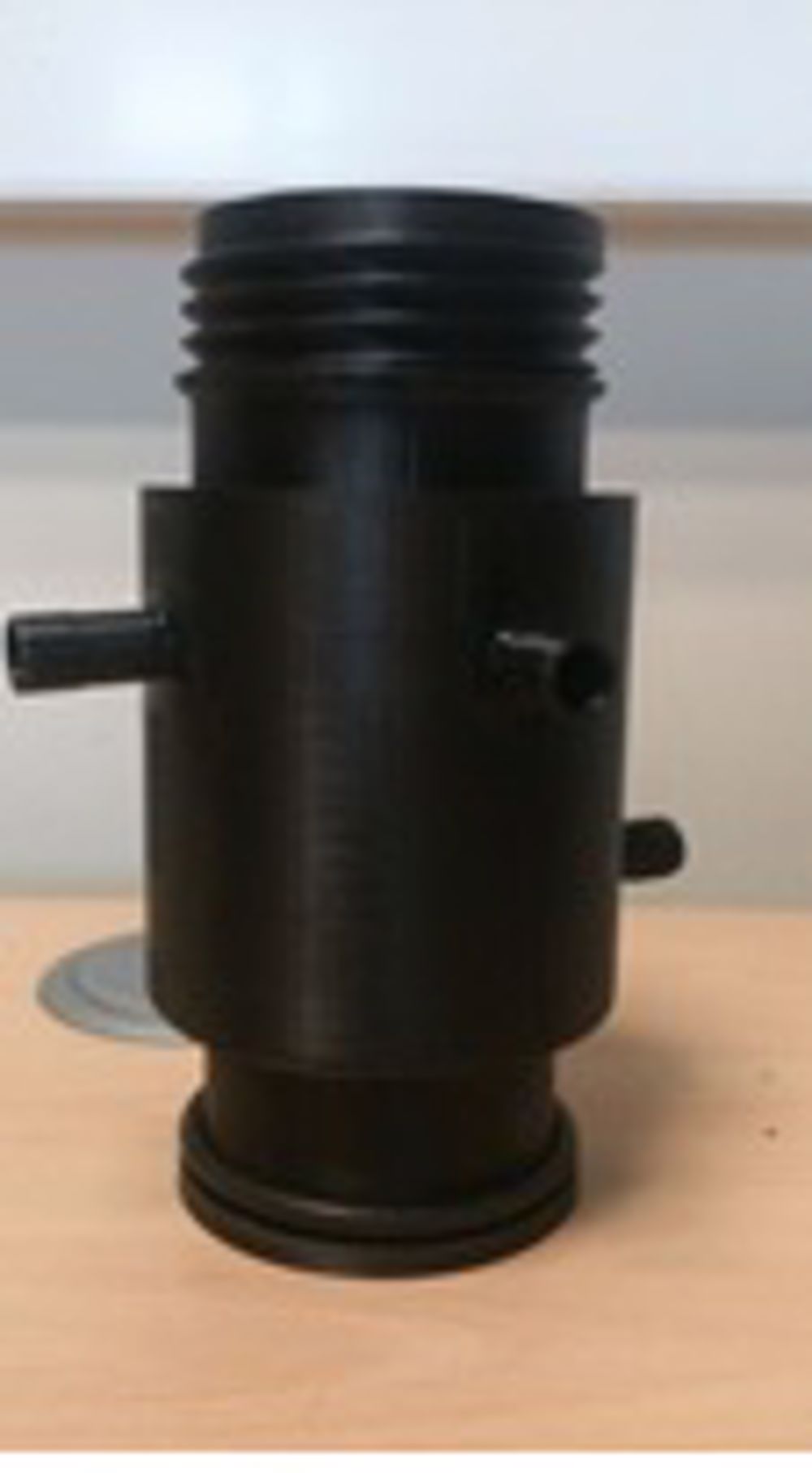
Fortunately, ultrasound (soundwaves with frequencies above 20 kHz - the limit of human hearing) can break down PFAS, into harmless inorganic species, including fluoride ions[16], CO2 and sulphate [20], in a process known as sonolysis[16]. Working with Jake Hurst of global environment and engineering consultancy Arcadis UK Ltd, we (Tim Sidnell and Madeleine Bussemaker) at The University of Surrey’s department of Chemical and Process Engineering are working to scale-up this technology to treat PFAS in firefighting foams, contaminated groundwater, wastewater and drinking waters. When applying ultrasound to aqueous PFAS, microscopic gas bubbles grow rapidly in size[17] and the hydrophobic PFAS tails orientate at the bubble interfaces. At a critical size, the bubbles violently implode, generating localised temperatures hotter than our sun’s surface and pressures of several hundred atmospheres[18,19], in a process similar to “cavitation pitting” seen in propellers and valves. A series of pyrolytic, radical and electron transfer mechanisms then occurs which breaks down the PFAS.
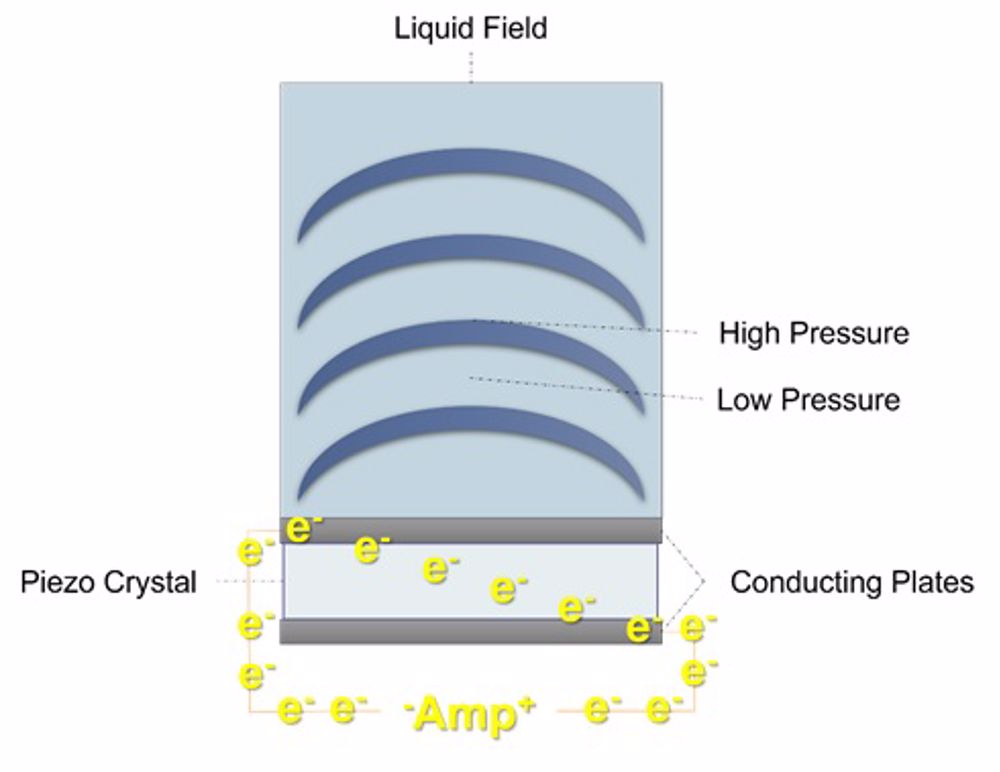
Our research necessitates a multidisciplinary approach across several universities and departments and incorporates reaction engineering with; electronics, soundwave physics, mass spectrometry, and environmental science. We probe the rate controlling conditions and reaction mechanisms using sonoluminescence (light emissions from collapsing bubbles) and sonochemiluminescence (luminol excitation by sonically generated radical species)[16]. To date, we’ve scaled our reactor volume over tenfold, with the potential to go even further in the future and developed a novel, simplified and faster PFAS quantification method, which we hope to publish soon.
Sonolysis is suited to low volume / high concentration waste streams, so it’s well coupled with traditional treatment technologies, such as membrane- or nanofiltration, or ion exchange resins, and is being tested alongside novel PFAS treatment technologies which Arcadis is developing (such as Ozofractionation). This enhances treatment efficiency and allows provision of potable water to millions with limited cost, chemical additives, or by-products. It’s our dream that ultrasonic reactors will soon be commonplace for PFAS treatment and in chemical engineering degree programs alike. So, in summary, we scream at dirty water to make it clean.
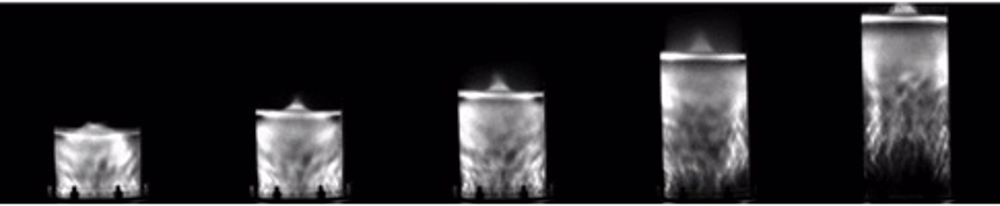
References
1. Ahrens, L. Polyfluoroalkyl compounds in the aquatic environment: A review of their occurrence and fate. J. Environ. Monit. 13, 20–31 (2011).
2. Buck, R. C. et al. Perfluoroalkyl and polyfluoroalkyl substances in the environment: Terminology, classification, and origins. Integr. Environ. Assess. Manag. 7, 513–541 (2011).
3. OECD. ENVIRONMENT DIRECTORATE JOINT MEETING OF THE CHEMICALS COMMITTEE AND THE WORKING PARTY ON CHEMICALS, PESTICIDES AND BIOTECHNOLOGY: TOWARD A NEW COMPREHENSIVE GLOBAL DATABASE OF PER- AND POLYFLUOROALKYL SUBSTANCES (PFASs): SUMMARY REPORT ON UPDATING THE OE. Ser. Risk Manag. No. 39., 1–24 (2018).
4. US EPA. PFAS Master List of PFAS Substances. USEPA Comptox Chemicals List (2020). Available at: https://comptox.epa.gov/dashboard/chemical_lists/pfasmaster.
5. 3M. The Science of Organic Fluorochemistry. 1–12 (1999).
6. O’Hagan, D. Understanding organofluorine chemistry. An introduction to the C-F bond. Chem. Soc. Rev. 37, 308–319 (2008).
7. Trier, X., Taxvig, C., Rosenmai, A. K. & Pedersen, G. A. PFAS in paper and board for food contact - options for risk management of poly- and perfluorinated substances. Nordic Council of Ministers 17, (2017).
8. Place, B. J. & Field, J. A. Identification of novel fluorochemicals in aqueous film-forming foams used by the US military. Environ. Sci. Technol. 46, 7120–7127 (2012).
9. Liu, C., Gin, K. Y. H., Chang, V. W. C., Goh, B. P. L. & Reinhard, M. Novel perspectives on the bioaccumulation of PFCs - The concentration dependency. Environ. Sci. Technol. 45, 9758–9764 (2011).
10. Gorrochategui, E., Pérez-Albaladejo, E., Casas, J., Lacorte, S. & Porte, C. Perfluorinated chemicals: Differential toxicity, inhibition of aromatase activity and alteration of cellular lipids in human placental cells. Toxicol. Appl. Pharmacol. 277, 124–130 (2014).
11. L. Wilder, R. Worley and Breysse, P. Community Exposures to Per- and Polyfluoroalkyl Substances in Drinking Water: A National Issue. J. Environ. Health 80, 38–41 (2016).
12. Harding-Marjanovic, K. C. et al. Aerobic Biotransformation of Fluorotelomer Thioether Amido Sulfonate (Lodyne) in AFFF-Amended Microcosms. Environ. Sci. Technol. 49, 7666–7674 (2015).
13. Tseng, N., Wang, N., Szostek, B. & Mahendra, S. Biotransformation of 6:2 Fluorotelomer alcohol (6:2 FTOH) by a wood-rotting fungus. Environ. Sci. Technol. 48, 4012–4020 (2014).
14. Rahman, M. F., Peldszus, S. & Anderson, W. B. Behaviour and fate of perfluoroalkyl and polyfluoroalkyl substances (PFASs) in drinking water treatment: A review. Water Res. 50, 318–340 (2014).
15. Chen, H., Zhang, C., Han, J., Yu, Y. & Zhang, P. PFOS and PFOA in influents, effluents, and biosolids of Chinese wastewater treatment plants and effluent-receiving marine environments. Environ. Pollut. 170, 26–31 (2012).
16. Wood, R. et al. Ultrasonic degradation of perfluorooctane sulfonic acid (PFOS) correlated with sonochemical and sonoluminescence characterisation. Ultrason. Sonochem. 68, 105196 (2020).
17. Okitsu, K. et al. Acoustic multibubble cavitation in water: A new aspect of the effect of a rare gas atmosphere on bubble temperature and its relevance to sonochemistry. J. Phys. Chem. B 110, 20081–20084 (2006).
18. Pflieger, R., Nikitenko, S. I., Cairós, C. & Mettin, R. Characterization of cavitation bubbles and sonoluminescence. (Springer, 2019). doi:10.1007/978-3-030-11717-7
19. Mason, T. J. & Lorimer, J. P. Applied Sonochemistry. Appl. Sonochemistry 2–3 (2002). doi:10.1002/352760054x
20. Vecitis, C. D., Park, H., Cheng, J., Mader, B. T. & Hoffmann, M. R. Kinetics and Mechanism of the Sonolytic Conversion of the Aqueous Perfluorinated Surfactants, Perfiuorooctanoate (PFOA), and Perfluorooctane Sulfonate (PFOS) into Inorganic Products. J. Phys. Chem. A 112, 4261–4270 (2008).